

The 3D knots were characterized by cryogenic transmission electronic microscopy (cryo-EM) single particle reconstruction, which confirmed their designated geometries. Furthermore, we expanded the design strategy to construct ssRNA knots and three-dimensional (3D) ssDNA knots.
#Single strand scaffold knot series
A series of design rules have been formulated to significantly improve the yield of well-formed target structures. The yield of the folded knots was optimized by programming a step-wise hierarchical folding pathway through sequence design in the paranemic cohesion regions. Various ssDNA knots that had different crossing numbers and that displayed two-dimensional (2D) patterns were designed, constructed, and characterized using atomic force microscope (AFM) imaging. Each of these ssDNA or ssRNA knots are folded and then self-assembled from a replicable single-stranded nucleic acid molecule with sizes ranging from 1700 to 7500 nucleotides (nt). A crossing number is a knot invariant that shows the smallest number of crossings in any diagram of the knot, representing the topological complexity of a knot 18. Here, we present a general approach to creating topological knots of ssDNA and ssRNA with high crossing numbers (up to 57). However, this strategy presents an important yet unanswered question: can partially paired, dsDNA thread through its own entanglement to form highly knotted topological structures? In contrast to the strategies that rely on the design of individual topological nodes and finding the appropriate DNA motifs/interactions with which to assemble such nodes, a different approach is to fold one long single-stranded DNA (ssDNA) chain into programmable topologies. Synthetic topological DNA nanostructures have previously been constructed by creating topological nodes based on B-form/Z-form double-stranded DNA (dsDNA) helices 11, 12, 13, 14, paranemic crossovers 15, 16, and DNA four-way junctions 17.

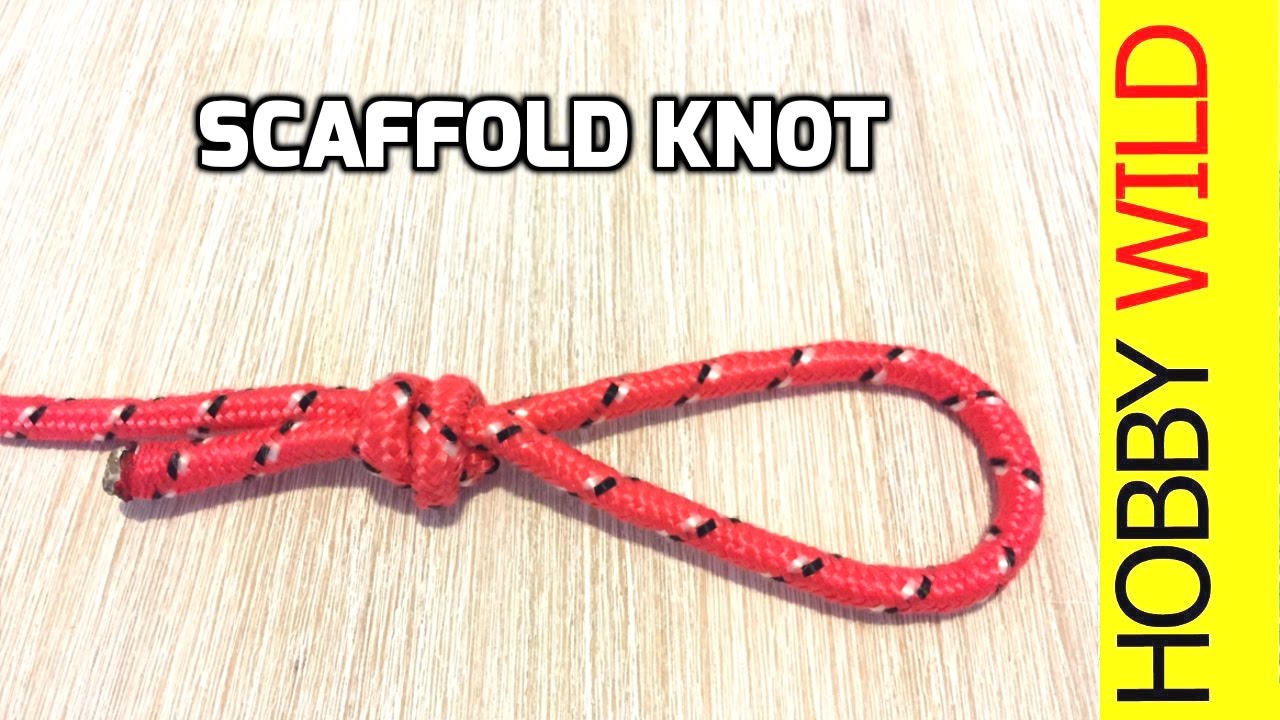
The programmability of nucleic acids 9, 10 makes them excellent candidates to use for creating topological knots at a molecular level. It is challenging to design and construct highly knotted nanostructures with well-defined topologies and geometries. The construction of molecular knots requires a high level of control over their assembly behaviors at a nanometer scale 8. Although knotted DNA structures are present in some bacterial phage genomes during the packing of the genomic DNA into the phage capsid 6, 7, they are not very predictable or well-organized. Knotted DNA structures are usually formed during genomic DNA replication and transcription and are eventually resolved by DNA topoisomerases 5. During protein folding, the protein molecules occasionally exhibit small amounts of crossings 1, 2, 3, 4. Naturally existing molecular knots have been found in biopolymers, such as DNA and proteins.
